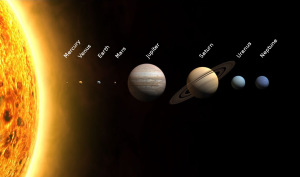
The 8 planets in our Solar System: four terrestrial planets close to the Sun, and four gas giants in the outer Solar System. (image: https://en.wikipedia.org/wiki/Solar_System)
The Sun’s planetary system, like the many other systems discovered in the last 2 decades, formed out of a protoplanetary disk. These disks are a natural by-product of star formation (almost every young star has one) and consist of a lot of gas (mostly hydrogen) and a small amount of solid dust particles. On timescales of hundreds of thousands of years, these dust grains collide and stick together, forming larger and larger bodies that eventually become asteroids, comets, and planetary embryos.
While the Sun’s protoplanetary disk is now long gone (disks typically dissipate on a timescale of a few million years), the planets hold clues to the disk’s properties. For example, the fact that the planets all lie in the same plane indicates that the disk was relatively flat, and the observation that the inner terrestrial planets are small and rocky while the outer planets are massive gas giants indicates that the snowline (inside of which water ice could not survive) was located somewhere between Mars and Jupiter. It is clear then that the properties of planets reflect, on some level, the environment in which they formed, and that understanding the physical and chemical structure of protoplanetary disks is a crucial step to understanding the ubiquity and diversity of planetary systems.
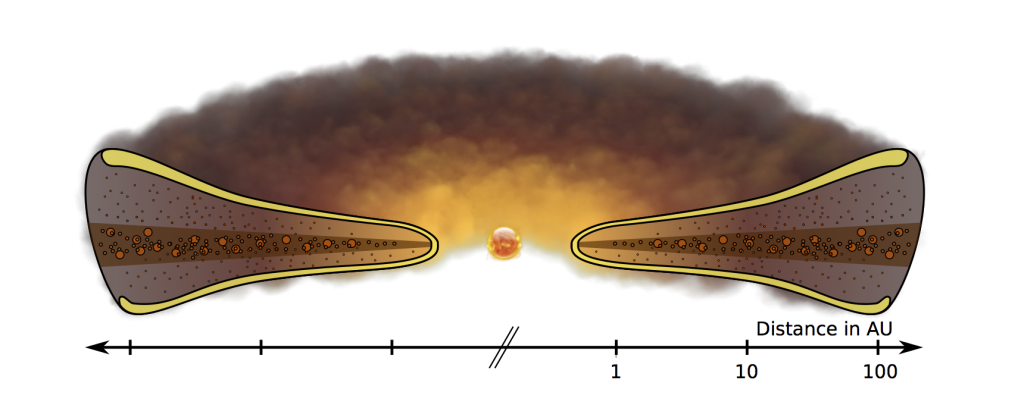
Illustration of a protoplanetary disk surrounding a young star. The larger dust particles are concentrated near the disk midplane, while the small grains remain well-mixed vertically. (image: http://www.til-birnstiel.de)
After moving to Chicago and becoming part of the EOS team in October 2015, I have been working on one question in particular: the vertical distribution of dust grains in planet-forming disks. It is usually assumed that, while small dust grains are well-mixed vertically, large grains are concentrated in the disk’s midplane. This picture comes from comparing the processes of gravitational settling (which acts to bring solids to the midplane) and turbulent diffusion (which tries to mix solids back up to the disk atmosphere). For large grains, settling is more efficient. For small grains, mixing is thought to win.
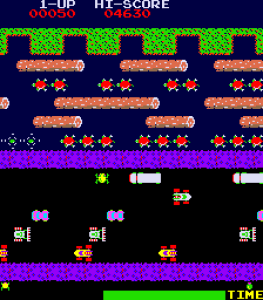
Frogger game screenshot. The frog in the bottom-left corner has to successfully navigate a busy road and a river filled with turtles and floating logs to reach his home in the top part of the screen. The frog’s journey resembles the process of vertical mixing of small grains in protoplanetary disks. (image: https://en.wikipedia.org/wiki/Frogger)
However, this approach neglects collisions between dust particles which might limit the mobility of the grains. In a way, it is like constructing an approach for crossing a busy road without taking into account collisions with passing cars (please don’t!). Continuing along these lines, a more accurate depiction of what happens when collisions are included is the classic video game Frogger (readers born in the 90’s or later are encouraged to play a few rounds before reading further). In the game, the player tries to navigate a frog (or, a dust grain) from one side of the road (the disk midplane) to the other (the disk atmosphere), while trying to avoid cars (other dust grains). Experienced players can tell you that successfully crossing the road becomes harder when the number of cars is increased, or when the frog moves much slower than the cars.
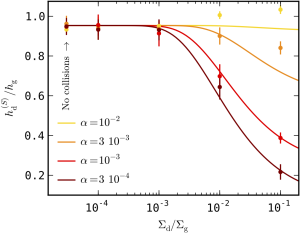
Relative scale-height of small grains in a protoplanetary disk. For low dust contents (left part of the plot) the scale-height is the same as that of the gas (relative scale-height of nearly 1). For high dust contents (right part of the plot) and low turbulence strengths (red and brown curves) the small dust becomes trapped in the midplane. (from Krijt & Ciesla 2016)
This is precisely what Fred Ciesla and myself found when simulating vertical diffusion/settling in the presence of collisions: efficient vertical mixing of small grains is not possible when the dust content is high, or when the turbulence is weak. This trapping of small grains could have important consequences for certain astro-chemical processes. For example, the surfaces of small grains play an important role in the formation and transport of water and other volatiles. Collaborating with Ted Bergin (University of Michigan), we are now beginning to couple dust evolution models to astro-chemical models in order to study how dust evolution influences the distribution of water vapor and water ice in planet-forming environments. Ultimately, this will contribute to our understanding of the formation of water-rich, possibly habitable planets. Stay tuned!
Read more:
Click here for the full paper on collisional trapping, which will appear in The Astrophysical Journal.